- Published 19 Feb 2025
- Last Modified 19 Feb 2025
- 13 min
Guide to Thermistors
This guide walks you through the function and purpose of thermistors, what they are made of and used for, and how to select a thermistor for your needs.

From the smartphones in our pockets to the appliances in our homes, thermistors play a hidden but crucial role in regulating temperatures. The name of these components is a portmanteau meaning “thermal resistor”. Unsurprisingly, they enable the precise control of heating systems, protect sensitive electronic components, and facilitate the efficient operation of devices.
In this comprehensive guide, we'll delve into the purpose of thermistors, how they function, various thermistor types, and their diverse applications across industries.
What is a Thermistor
A thermistor is a type of resistor that is particularly sensitive to changes in temperature. Unlike standard resistors, which have a relatively stable resistance, a thermistor's resistance changes significantly as the temperature fluctuates.
This unique characteristic makes thermistors invaluable for temperature measurement and control in electronic devices and systems. They are commonly used in everything from consumer electronics and automotive systems to industrial equipment and medical devices.
What Does a Thermistor Do?
A thermistor's primary function is to sense and respond to changes in temperature. As the temperature rises, the resistance of a thermistor will either increase or decrease, depending on its temperature coefficient.
Most thermistors have a negative temperature coefficient (NTC), meaning their resistance decreases as the temperature increases. This change in resistance can be used to measure temperature or to control circuits and devices in response to temperature variations.
How Does a Thermistor Work?
The working principle of a thermistor is based on the relationship between temperature and electrical resistance. When the temperature of a thermistor changes, the movement of electrons within its material is affected, leading to a change in its electrical resistance.
The change in resistance can be measured and correlated to the temperature, allowing thermistors to function as accurate temperature sensors. Moreover, the change in resistance can be used to trigger other components in a circuit, such as switches or safety relays, enabling temperature-dependent control of various devices and systems.
How Does a Thermistor Work in a Controlled System?
A controlled system, in the context of electronics, refers to a system that maintains a specific parameter, such as temperature, within a desired range. This control is achieved through a feedback loop that continuously monitors the parameter and adjusts the system's output accordingly.
Thermistors are key components in such systems, acting as the temperature-sensing element that provides feedback to the control mechanism. For example, precise temperature control is crucial for the print bed and extruder nozzle of a 3D printer. A thermistor attached to the print bed acts as the system's "eyes" to monitor the bed's temperature. As the temperature fluctuates, the thermistor's resistance changes, generating a signal that is sent to the 3D printer's control board.
The control board then compares this signal to the desired temperature setpoint and adjusts the power supplied to the heater to maintain the optimal temperature. A heatsink may be used to help with heat dissipation. This precise control ensures that the printing material is heated correctly for extrusion and that the print bed is at the ideal temperature for adhesion.
The Feedback Loop
The feedback loop involves measuring temperature, comparing it to the setpoint, and adjusting the system to maintain stability. Thermistors play a crucial role in this process by providing accurate temperature data, enabling precise control.
Importance of Thermistor Placement
Proper placement ensures accurate and stable temperature measurement. The thermistor should be positioned close to the target area to reduce thermal lag. To prevent self-heating, engineers may select low-resistance thermistors or optimise bias currents.
In complex systems, multiple thermistors can monitor different areas, providing a comprehensive view. For example, in refrigeration, multiple sensors ensure consistent cooling throughout the compartment.
Configuring the Control System
Once the thermistor is placed, system configuration includes selecting the base resistance (typically at 25°C), setting bias current, and calibrating the setpoint temperature. These steps ensure reliable and precise temperature control in a variety of applications.
What Are Thermistors Made Of?
Thermistors are typically constructed from a combination of metal oxides, which are materials that exhibit a change in electrical resistance with temperature. The specific composition of these metal oxides varies depending on the type of thermistor and its intended application. Here are some common metals used in thermistor construction:
- Copper: Copper is a highly conductive metal often used in thermistors to enhance their electrical conductivity. Its presence helps to ensure efficient transfer of electrical signals and minimise resistance, contributing to the overall performance of the thermistor.
- Nickel: Nickel is a versatile metal with good electrical conductivity and resistance to corrosion. In thermistors, nickel can be used to improve stability and enhance performance across a wider temperature range.
- Manganese: Manganese oxides are commonly used in Negative Temperature Coefficient (NTC) thermistors due to their significant negative temperature coefficient. The presence of manganese contributes to the thermistor's ability to exhibit a large decrease in resistance as temperature increases.
- Cobalt: Cobalt oxides are another common component in NTC thermistors. They contribute to the thermistor's sensitivity to temperature changes and enhance its ability to provide accurate temperature readings.
- Barium: Barium titanate is a ceramic material often used in Positive Temperature Coefficient (PTC) thermistors. It exhibits a positive temperature coefficient, meaning its resistance increases with temperature. This property makes barium titanate useful for applications such as overcurrent protection.
- Titanium: Titanium dioxide is a versatile material with various applications in electronics. In thermistors, titanium dioxide can be used to improve stability, enhance temperature sensitivity, and tailor the thermistor's resistance-temperature characteristics.
The specific combination and proportions of these materials determine the thermistor's overall performance characteristics, including its temperature coefficient, resistance range, and operating temperature range.
Types of Thermistors
Thermistors are broadly categorised into two main types based on their temperature coefficient: Negative Temperature Coefficient (NTC) and Positive Temperature Coefficient (PTC). Additionally, thermistor types also differ by their resistance-temperature curves and their specific application ranges.
Negative Temperature Coefficients (NTC Thermistors)
NTC thermistors are the most common type of thermistor. Their resistance decreases as the temperature increases, making them suitable for a wide range of temperature sensing and control functions.
NTC thermistors are typically made from semiconductor materials like metal oxides, and they are available in various forms, including beads, discs, and chips. They are widely used in applications such as temperature measurement, temperature compensation, and inrush current limiting.
Positive Temperature Coefficients (PTC Thermistors)
PTC thermistors exhibit the opposite behavior of NTC thermistors, with their resistance increasing as the temperature rises. This characteristic makes them useful for applications such as overcurrent protection, self-regulating heating, and liquid level sensing. PTC thermistors are often used in circuits as resettable fuses, protecting against overcurrent conditions.
10K Thermistor
A 10K thermistor is a specific type of NTC thermistor with a resistance of 10,000 ohms at 25°C. This is a common value used in various applications, including temperature sensing in electronic circuits, automotive systems, and industrial equipment. 10K thermistors offer good accuracy and stability over a moderate temperature range.
3K Thermistor
A 3K thermistor is another type of NTC thermistor with a resistance of 3,000 ohms at 25°C. These thermistors are often used in applications where a lower resistance value is desired, such as in temperature sensing for low-power circuits or in applications with higher current requirements.
12K Thermistor
A 12K thermistor is an NTC thermistor with a resistance of 12,000 ohms at 25°C. These thermistors are often used in applications where a slightly higher resistance value is preferred, such as in temperature sensing for specific electronic circuits or in applications with lower current requirements.
Epoxy-Coated Thermistor
Epoxy-coated thermistors are encapsulated in a protective epoxy coating, providing durability and resistance to moisture and other environmental factors. They are suitable for applications where the thermistor may be exposed to harsh conditions or where protection from mechanical damage is required.
Glass-Coated Thermistor
Glass-coated thermistors are encapsulated in a glass coating, offering excellent stability and resistance to high temperatures and corrosive environments. They are often used in applications where the thermistor needs to withstand extreme temperatures or harsh chemicals.
Thermistor Electrical Circuit Symbol
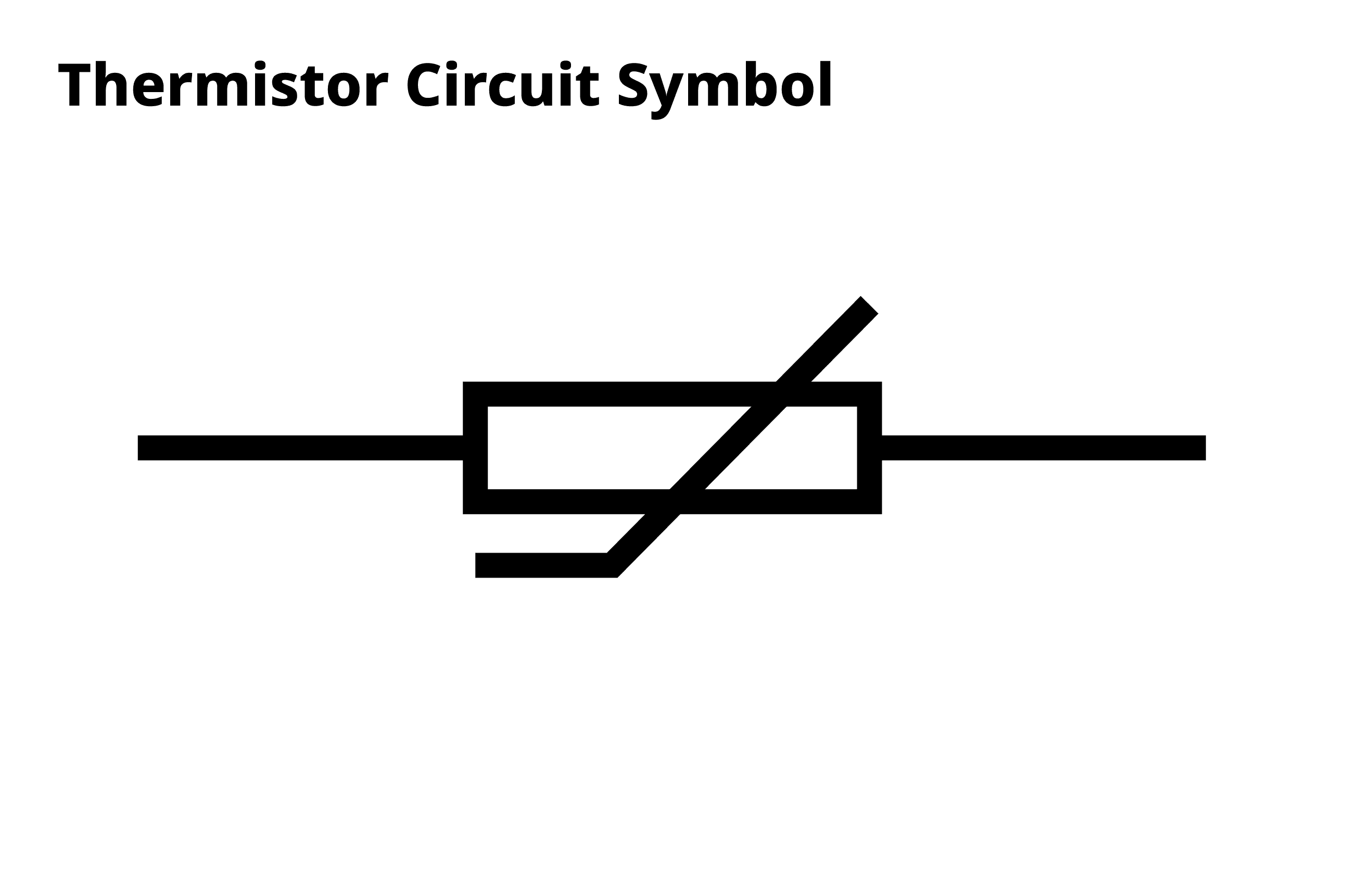
Like other electronic components, thermistors have standardised symbols that represent them in circuit diagrams. According to the IEC (International Electrotechnical Commission) standard, the thermistor symbol consists of a rectangle with a diagonal line through it, indicating its variable resistance.
Variations of this symbol may include a 't°' to signify temperature dependence or an arrow to indicate the direction of resistance change with temperature. However, these additions are not always included.
These symbols are essential for clear communication and understanding of circuit diagrams, enabling engineers and technicians to quickly identify and interpret the function of thermistors within a circuit. They provide a concise and standardised way to represent these temperature-sensitive components, facilitating circuit design, analysis, and troubleshooting.
Advantages and Disadvantages of Thermistors
Advantages
- Sensitivity to temperature: Thermistors exhibit a high degree of sensitivity to temperature changes, meaning their resistance changes significantly even with small temperature variations. This sensitivity makes them ideal for precise temperature measurement and control.
- Low cost: Thermistors are relatively inexpensive compared to other temperature-sensing devices, making them an attractive option for cost-sensitive applications.
- Small size: Thermistors are available in very small sizes, making them suitable for applications where space is limited, such as in miniature electronic devices or compact sensors.
- Compact: Their compact size and simple construction make them easy to integrate into various devices and systems without adding significant bulk or complexity
Disadvantages
- Non-linear: The relationship between resistance and temperature in thermistors is non-linear, meaning their resistance does not change proportionally with temperature. This non-linearity can make calibration and signal processing more complex.
- Limited operating range: Thermistors typically have a limited operating temperature range, performing best within a specific temperature range around their base resistance. Outside this range, their accuracy may decrease.
- Require excitation current: Thermistors require a small excitation current, or voltage source, to operate, which can generate some self-heating and potentially affect the accuracy of the temperature measurement.
- Limited accuracy: While thermistors offer good accuracy for many purposes, their accuracy can be limited compared to other temperature sensors, such as thermocouples or resistance thermometers, particularly at extreme temperatures.
What are Thermistors Used For?
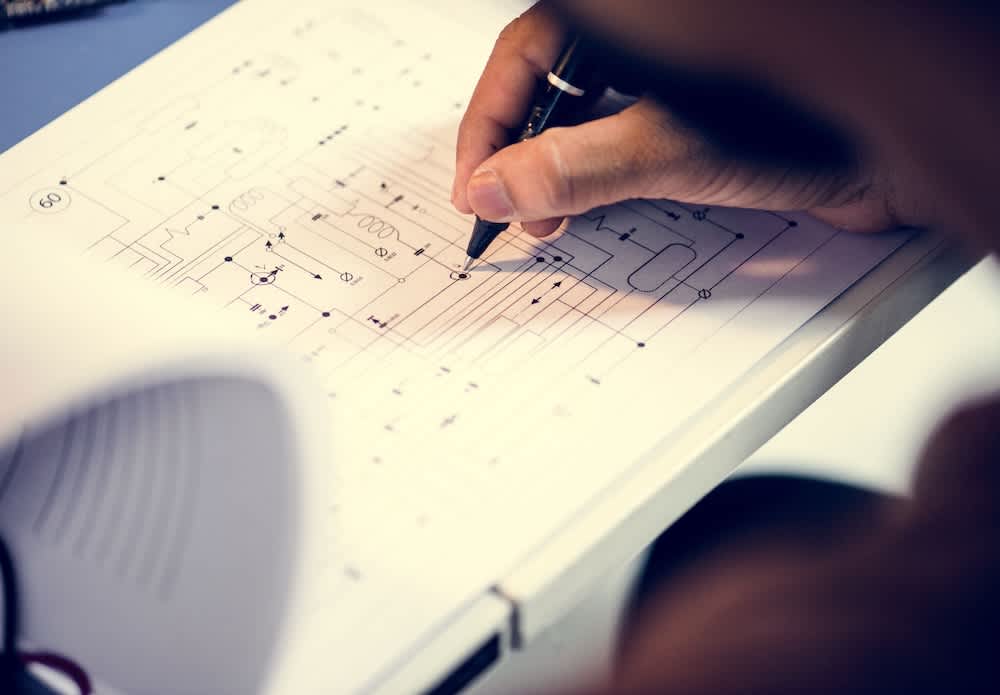
Thermistors are versatile temperature sensing devices that find applications across a wide range of industries and products, such as:
- White goods: Thermistors are used in various household appliances, such as refrigerators, washing machines, and ovens, to monitor and control temperature. For example, in a refrigerator, a thermistor senses the temperature inside the compartment and sends a signal to the control unit to regulate the cooling system.
- Manufacturing facilities: Many industrial processes rely on thermistors to monitor and control temperatures in machinery, equipment, and production lines. For instance, in a plastics manufacturing plant, thermistors might be used to monitor the temperature of extruders and injection moulding machines, ensuring optimal processing conditions.
- 3D printing: As essential components in 3D printers, thermistors provide precise temperature control of the print bed and extruder nozzle to ensure optimal printing conditions. They help to maintain the correct temperature for the printing material, ensuring proper adhesion and preventing warping or other printing defects.
- Medical applications: Thermistors are used in various medical devices and equipment, such as digital thermometers, patient monitoring systems, and medical imaging equipment.
- Food and beverage: Used in the food and beverage industry, thermistors monitor and control temperatures during processing, storage, and transportation of food products. For instance, thermistors can be used to monitor the temperature of food storage warehouses, ensuring that perishable goods are kept at the correct temperature to maintain quality and safety.
- Temperature sensors: Thermistors are widely used as temperature sensors in various applications, including automotive temperature sensing, HVAC systems, and scientific instruments.
- Computers: A crucial component in computers and other electronic devices, thermistors monitor temperature and prevent overheating. They are often found in CPUs, power supplies, and other components that generate heat, ensuring that the devices operate within safe temperature limits.
How to Choose a Thermistor
RS Australia sources its thermistors from brands like EPCOS, TE Connectivity, and Amphenol Advanced Sensors, featuring various models and materials.
With this variety, selecting the right thermistor for your purposes depends on several parameters related to its intended use and performance requirements. Here are some key factors to consider:
- Bill-of-materials (BOM) cost: Consider the overall cost of the thermistor and any associated components, such as resistors or amplifiers, that may be required for your application. For example, if you need to linearise the output of an NTC thermistor, you may need to add extra resistors, which can increase the BOM cost.
- Resistance tolerance: Resistance tolerance indicates the potential variation in resistance at a given temperature, so choose a thermistor with a resistance tolerance that meets your accuracy requirements. For instance, if you need precise temperature measurement, a thermistor with a lower tolerance would be preferred.
- Calibration points: The number of calibration points required for a thermistor depends on the temperature range and accuracy needs of your application. For wider temperature ranges or higher accuracy, more calibration points may be necessary.
- Sensitivity to temperature: The sensitivity of a thermistor refers to how much its resistance changes per degree Celsius. Choose a thermistor with a sensitivity that is appropriate for your application. For instance, if you need to detect small temperature changes, a thermistor with higher sensitivity would be beneficial.
- Self-heating and sensor drift: Thermistors generate a small amount of heat when current flows through them, which can affect the accuracy of the temperature measurement. Additionally, sensor drift, which refers to changes in the thermistor's resistance over time, can also affect accuracy. Look for thermistors with low sensor drift for long-term stability.
Calibrating a Thermistor
Calibrating a thermistor involves comparing its readings to those of a known, accurate temperature standard. This process helps to identify and correct any deviations in the thermistor's response, ensuring that it provides reliable and precise temperature measurements.
- Prepare the equipment: Gather the necessary equipment, including the thermistor to be calibrated, a reference thermometer with known accuracy, a temperature-controlled environment (such as a water bath or oven), and a multimeter to measure the thermistor's resistance.
- Stabilise the temperature: Set the temperature-controlled environment to a specific temperature within the thermistor's operating range. Allow sufficient time for the temperature to stabilise.
- Measure the reference temperature: Use the reference thermometer to accurately measure the temperature of the environment.
- Measure the thermistor's resistance: Connect the multimeter to the thermistor and measure its resistance at the stabilised temperature.
- Record the data: Record the reference temperature and the corresponding thermistor resistance.
- Repeat at different temperatures: Repeat steps 2 to 5 at several different temperatures within the thermistor's operating range. This will provide a set of data points that can be used to create a calibration curve or equation.
- Derive calibration curve: The curve or equation can then be used to convert the thermistor's resistance readings into accurate temperature values.